Differences in Vaccine and SARS-CoV-2 Replication Derived mRNA: Implications for Cell Biology and Future Disease
Kevin McKernan1, Anthony M. Kyriakopoulos2, Peter McCullough3
Full Length Version on OSF.io
1.Medicinal Genomics, Beverly Mass, USA. Kevin.McKernan@medicinalgenomics.com
2.Nasco A.D. Biotechnology Laboratory, 11 Sacthouri Str. 18536 Piraeus, Greece.
3. Truth for Health Foundation, Tucson, AZ, USA
Abstract
Codon optimization describes the process used to increase protein production by use of alternative but synonymous codon changes. In SARS-CoV-2 mRNA vaccines codon optimizations can result in differential secondary conformations that inevitably affect a protein’s function with significant consequences to the cell. Importantly, when codon optimization increases the GC content of synthetic mRNAs, there can be an inevitable enrichment of G-quartets which potentially form G-quadruplex structures. The emerging G-quadruplexes are favorable binding sites of RNA binding proteins like helicases that inevitably affect epigenetic reprogramming of the cell by altering transcription, translation and replication. In this study, we performed a RNAfold analysis to investigate alterations in secondary structures of mRNAs in SARS-CoV-2 vaccines due to codon optimization. We show a significant increase in the GC content of mRNAs in vaccines as compared to native SARS-CoV-2 RNA sequences encoding the spike protein. As the GC enrichment leads to more G-quadruplex structure formations, these may contribute to potential pathological processes initiated by SARS-CoV-2 molecular vaccination.
Introduction
The simplification of scientific jargon in the realm of public health can lead to the construction of a false consensus. One such over-simplification exists in our discussions surrounding the expression of SARs-CoV-2 spike protein in mRNA vaccines. This spike protein is often referred to as being bio-equivalent to the naturally expressed spike protein in SARs-CoV-2. Accordingly, it is suggested that this "may” constitute a safer immunological exposure as the rest of the genes responsible for replication of the virus are omitted. This often leads one to assume that the pathologies that arise from vaccine expressed spike protein should be a subset of those you might experience with the full-length live virus. The mRNA vaccines have the benefit of being a non-replication competent immune exposure, but are the spike proteins truly equivalent?
In this line of questioning, one must ask what is the purpose for codon optimizing a viral mRNA that is already adapted to its host? This does not come risk free. The potential dangers of codon optimization have been raised for in vivoapplications 1. Even synonymous codon changes incorporated into mRNA vaccines can alter the expected encoded protein conformation as the translation speed and efficiency can result in different protein folding. Despite identical amino acids, the altered conformation can function differently as compared to synonymous codon replacements of native mRNAs that have been put in place under the selective pressure of evolution of parasite-host adaptation. Codon optimization strategies for the development of mRNA vaccines can result in immune de-regularities, affect epi-transcriptomic regulation, and can lead to disease progression 1 2.
Methods
We used open source, publicly available software for every step of the analysis.
Sequences utilized
Vaccine derived mRNAs were downloaded from Dae Eun Jeong et al.
Using BLAST of the Wuhan Hu-1 reference sequence against the vaccine derived RNAs, we extracted the Wuhan Hu-1 spike sequence. Query13637:21560-25382 NC_045512.2 Severe acute respiratory syndrome coronavirus 2 isolate Wuhan-Hu-1, complete genome
GC content
GC content of each sequence was calculated3. Biologicscorp.https://www.biologicscorp.com/tools/GCContent/
RNAfold analysis
Using default conditions from a tool known as RNAfold, secondary structures were predicted4. http://rna.tbi.univie.ac.at/cgi-bin/RNAWebSuite/RNAfold.cgi
G4 Identification
We used QGRSMapper to calculate G4 motifs in the three mMRNA sequences.https://bioinformatics.ramapo.edu/QGRS/index.php5
Frameshift evaluation
We used IGV2.4.16 to visualize all 6 reading frames of the 5’ UTR6-8.
Results and Discussion
To address the implied equivalency of virus derived spike protein vs mRNA derived spike protein, we explore the impact of codon optimization on the secondary structure of the natural RNA encoded spike protein and compare this to the secondary structure of the mRNA vaccines. The most obvious alteration from codon optimization is the increase in the GC content of the mRNAs (Figure 1.)
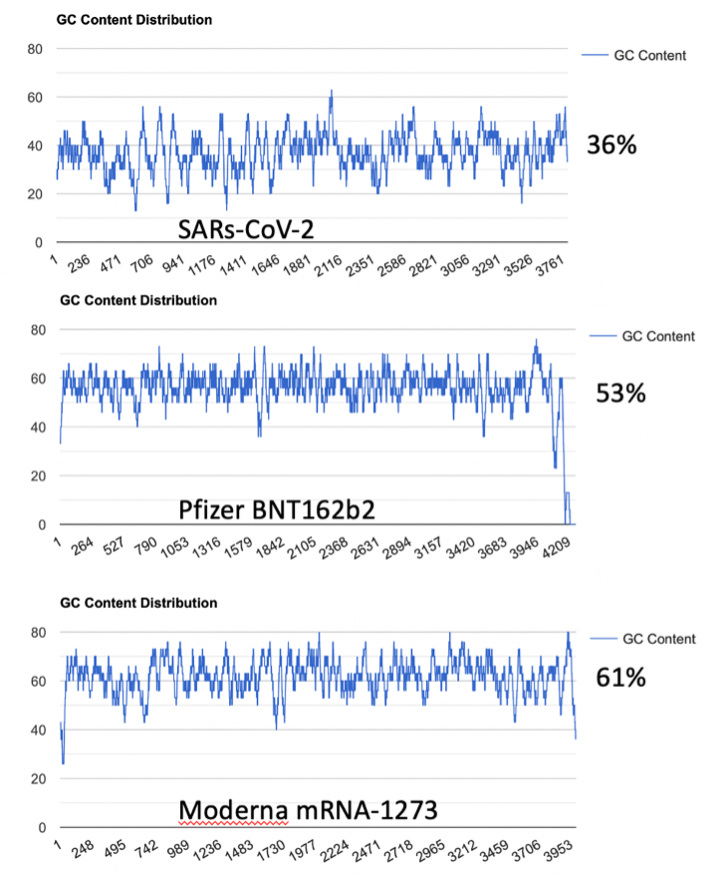
This increased GC content significantly alters mRNA secondary structure. Using RNAfold (http://rna.tbi.univie.ac.at/cgi-bin/RNAWebSuite/RNAfold.cgi) we see the changes to secondary structure in the vaccine derived mRNAs compared to the native virus (Figure 2)4. This is a result of codon optimization that was likely performed without the consideration of secondary structures like quadruplex G formation.
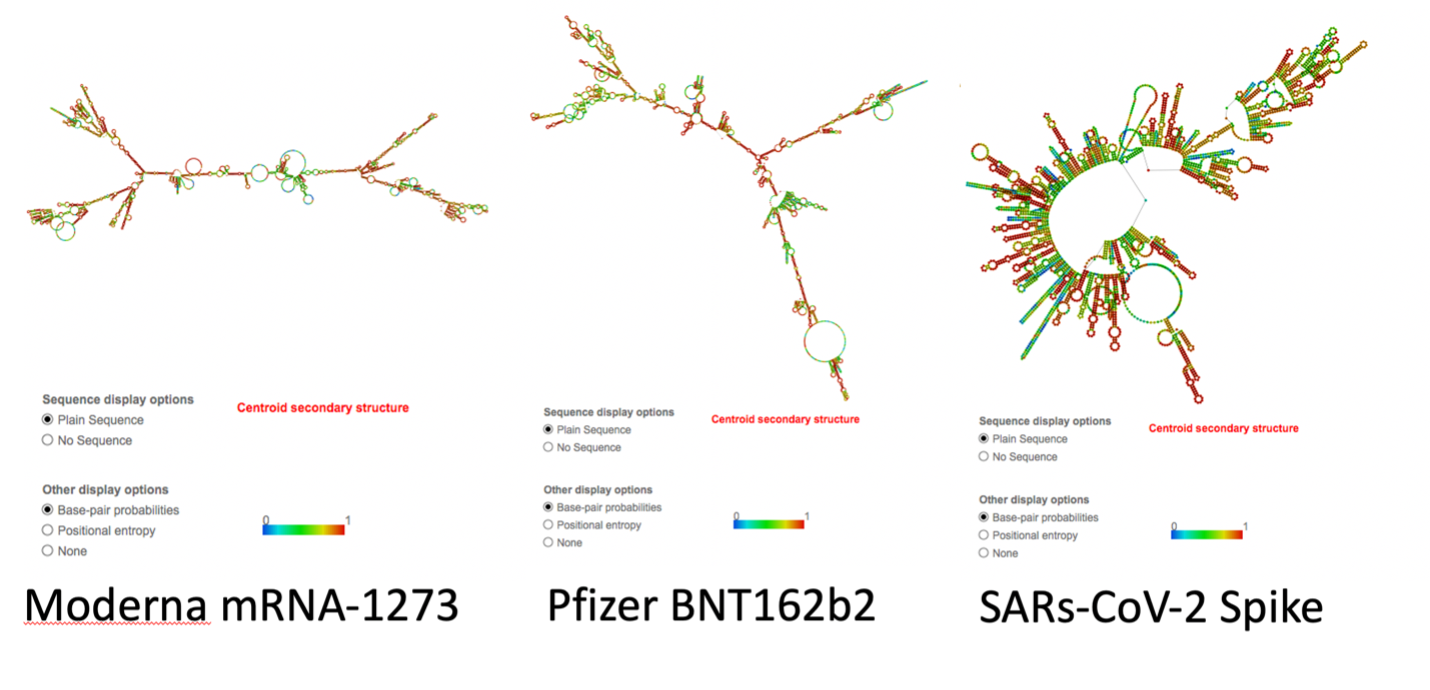
Not only are the secondary structures of the mRNA noticeably different, of note, is the increased number of quadruplex G formations in the codon optimized mRNA vaccines (Figure 3). Quadruplex G formations (G4s) in SARs-CoV-2 are highly conserved across over 16,466 SARs-CoV-2 genome sequences 9. They are believed to play a critical role in transcriptionand translation of SARs-CoV-2 peptides 10-12. G4 formations in the RNA sequence for nucleocapsid protein have been proposed as attractive drug targets to eliminate nucleocapsid expression. This is achieved by using compounds that stabilize quadruplex G formations 13.
G4s are also implicated in recruiting viral SARs Unique Domain (SUD) of Nsp3 14. While the mRNA vaccines do not encode this non-structural protein, an increasing number of breakthrough infections must consider the biology of both the virus and the non-native vaccine derived mRNA. With increased vaccination rates and continued boosters, it will be increasingly important to understand the immune status of patients expressing both mRNA-based vaccine spike proteins concurrent with viral spike protein expression.
The changes to secondary structure can be observed in the G4 formations seen in each sequence using QGRSMapper (Figure 3) 5. Similar trends were observed using G4-iM Grinder (Personal communication Belmonte)12.
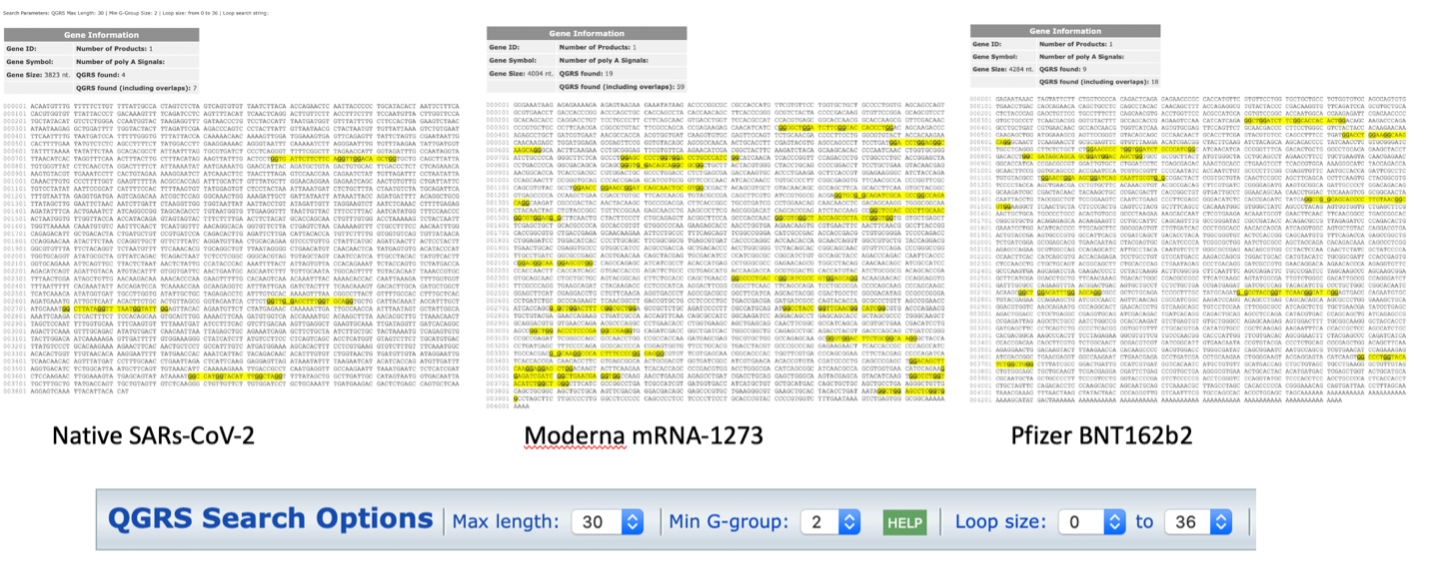
Does the increase of 4 to 19 G4 motifs alter the translation efficiency of these proteins? Do they create truncated spike proteins not seen with the natural virus?
Fidelity of Spike protein expression
To address these questions, there is another unique feature of the mRNA vaccines that must be considered. The uracil’s in the vaccines have been replaced with N1-methylpseudouridine. The use of N1-methylpseudouridine in these mRNAs will further complicate the folding predictions as N1-methylpseudouridine (m1Ψ) has promiscuous base pairing with G and A and is known to create errors in translation 15-17. These m1Ψ replacements are excellent for evading host RNAses but they are also implicated in Toll Like Receptor biology and this is something unique to mRNA vaccines. While m1Ψenables slower degradation of mRNAs, it comes at the cost of camouflaging the mRNA from the immune system that targets viral RNA secondary structures 18. Immune receptor TLR3,7,8 and RIG-1 are intimately involved in targeting such secondary structures and their response is RNA modification dependent 19. This has been described as “reprogramming the innate immune and adaptive immune response” 20. This may explain the increased rate of Herpes Zoster, HHV6/HHV7 (Pityriasis rosea) and Epstein-Barr reactivation post mRNA vaccination 21-26. These reactivations are also seen with SARs-CoV-2 infection. Further investigation is required to understand if the mRNA vaccines exacerbate or curtail these reactivations.
Codon optimizations and pseudouridine replacements alter the secondary structure significantly and likely change the Toll Like Receptor activity one might find with the native virus 27. How these receptors behave in the presence of both vaccine-derived spike mRNA and viral derived spike mRNAs is a nascent field. However, this field is of interest to many physicians concerned about chronic diseases including cancer 28. Jiang et al. note that the spike protein localizes to the nucleus and significantly alters DNA damage and repair pathways via modified VDJ recombination required for adaptive immunity 29. Many of these vaccine trials demonstrated lymophocytopenia and neutropenia 2 weeks after injection 30 31. Down regulation of the innate immune system concurrent with reduced DNA repair may lead to carcinogenesis32.
Notably, promiscuous bases like inosine and pseudouridine are known to stabilize quadruplex Gs 33 34 further exacerbating the impact of G4 quartet formation with codon optimization.
How much m1Ψ is present in these mRNAs? The only independent DNA sequence released for these mRNA vaccines were sequenced on Illumina platforms that are blind to pseudouridine 35. The exact m1Ψ density (while reported by the manufacturers to be 100%) has not been independently sequence verified. The most parsimonious synthesis approach described by Nance et al. replaces all uracil’s with m1Ψ via polymerase incorporation 18. Such an approach would also leave the stop codons prone to pseudouracil stop codon read through described by Fernandez et al. 36. Pseudouridine is also known to create ribosomal frameshifts. Penultimate to the ΨGA ΨGA stop codon in the BNT162b2 vaccine is an out of frame human amino acid sequence of unknown function (AAG23172.1) (Figure 4). Xia et al. also makes note that UGA stop codons are more prone to read through and +1 frameshifts suggesting these mRNA derived stop codons may not be as effective as viral derived stop codons 15.
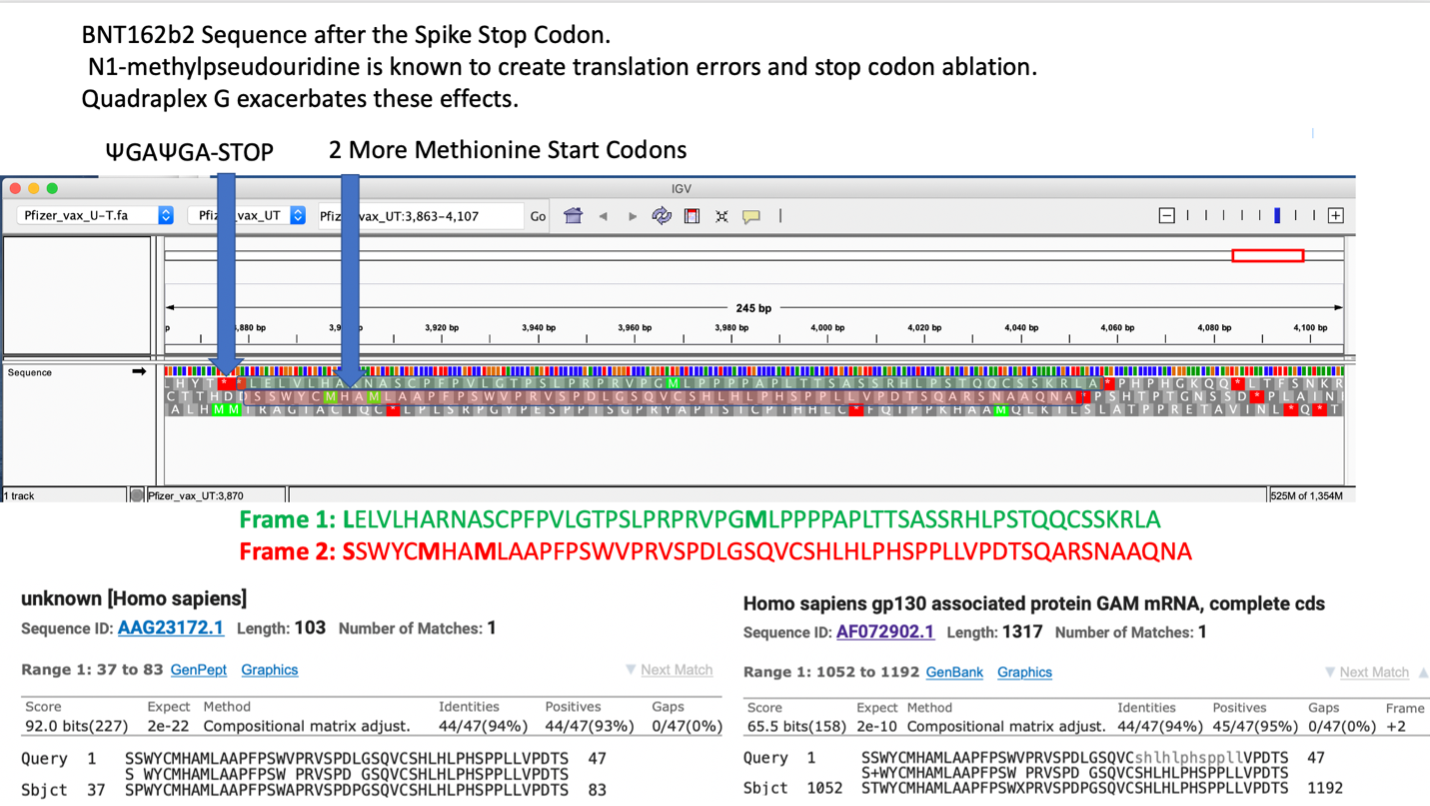
Expression of chimeric spike-human peptides may be unique to pseudouracil based mRNA vaccination and raises concerns over immune de-regulation including autoimmunity that may develop with such chimeric inoculations.
Hypothetical viral: mRNA vaccine interactions
With any virus or vaccine that enables latent virus reactivation we must consider the case of viral recombination. Chimeric RNAs are more likely to form with mRNAs that have degenerate bases. In this hypothetical case, a non-replicative pseudouridylated mRNA may hitchhike into a replication form via recombination with a live virus.
Relevantly, in vitro and in vivo experiments using human and mouse RNAse L chimeras, and chimeric Mouse Hepatitis Virus (coronavirus) expressing recombinant L* from Theiler's murine encephalomyelitis virus (picornavirus) showed that chimeric MHV viruses are functional. These efficiently express RNAse L inhibitors and hence interfered with prompt interferon responses37. Linking to SARs-CoV-2, the subunit 1 (S1) persists for up to 15 months post infection and is related to post-acute inflammatory sequalae presenting with neurological complications38. 75% of Patients with persistent RNAemia also present long COVID39.
Retroviral and non-retroviral RNA sequences are abundantly integrated into mammalian DNA40. These inserted viral sequences exist in the form of long interspersed nuclear elements (LINEs). These LINE based retrotransposons mobilize and also transcribe human DNA not associated with the LINE sequences forming pseudogenes 41. These can be active in disease onset including tumorigenesis 42. As an example, the polio RNA sequences are identified with a 100 % sequence homology to human chromosomes and are associated with cancer progression43. These require attention for possible production of chimeric mRNAs with SARS-CoV-2 native or vaccine derived mRNAs, especially during meiotic division44, stem cell differentiation and cancer45.
Furthermore, human endogenous retroviruses (HERVs) constitute the 8% of human DNA. These are mobile genetic elements related to etiopathogenesis of inflammation and neurogenerative disease40. Due to viral transactivation of HERVs the herpesviridae reactivation is implicated in the onset of multiple sclerosis 46. Another example of reactivation disease is Ebstein Barr virus associated head and neck cancer. Notably, HERVs induce neurological autoimmunity by interfering with innate immunity in mice 47.
Moreover, the presence of chimeric SARS-CoV-2 mRNAs with other viral sequences has recently been reported 48 49. Other studies describe a decrease in the interferon response50 51 and emergence of Herpes Zoster Virus infection post SARS-CoV-2 molecular vaccination23 21
It is important to understand these are not documented cases of SARs-CoV-2 viral recombination but such hypothetical cases resonate with recently published clinical data52. Likewise, virus to mRNA recombinations increase in likelihood with mRNAs that use degenerate bases like m1Ψ53. Notably, the natural Ψ:U ratio of mRNAs under normal conditions is markedly low (0.2-0.6 %) compared to the 100% Ψ substitutions in synthetic SARS-CoV-2 mRNAs54. RNA based Pseudouridine substitutions determine the cell’s fate and differentiation55. Specifically, pseudouridylations of mRNAs are subject to activity of PUS7 writer protein which is a key regulator of protein translation and determinant of stem cell growth and differentiation55. Dysregulation of PUS7 activity correlates with agitated protein synthesis in stem cells which leads to hematological disorders and aggressive stem cell acute myeloid leukemia56. Also pertinent to the detection of the SARs-CoV-2 recombination hypothesis is that many SARs-CoV-2 sequencing methods (ARTIC) rely on SARs-CoV-2 specific PCR primers that are often blind to recombination events 57. Given the paucity of sequence information on the vaccines and the peptides expressed in-vivo with such mRNAs, further work is required to confirm pseudouridine induced promiscuous translation or viral recombination with mRNA vaccines.
Limited evidence of pure vaccine derived spike protein expression in humans
There is limited literature describing fidelity of mRNA vaccine derived spike protein expression. The best in-vivoevidence of heterogeneous translation of vaccine derived spike protein is from Figure 2C in Bansal et al. where the SDS-PAGE of vaccinated patients’ exosome-derived spike proteins exhibit broad banding patterns (Figure 5). To rule out electrophoretic artifacts from membrane derived proteins, LS/MS-MS should be performed to confirm or negate the spike translation heterogeneity. Additionally, spike proteins expressed from a DNA based plasmid in HEK293T cells described by Jiang et al. demonstrate 3 different bands on SDS-PAGE. These 3 bands represent the glycosylated, full length and fragmented spike proteins and their expression appears cell fraction dependent. DNA based expression does not contain error prone pseudouridine and one would expect more translational error from the m1Ψ mRNA-based vaccine expression systems.
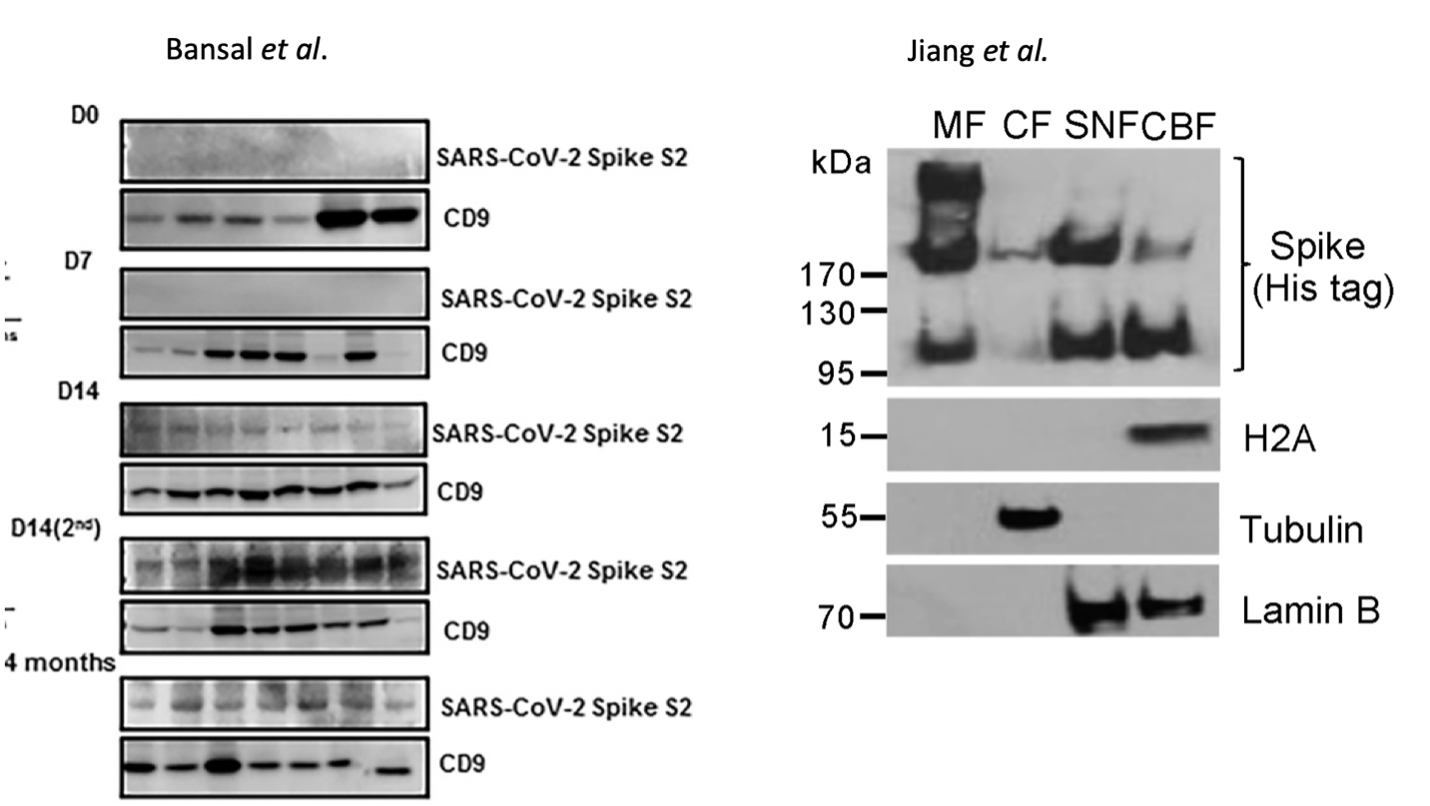
While these truncated or elongated promiscuous translation products may be rare events, this does bring to focus the question of dosage and spike protein duration with mRNA vaccines that ablate many components of the innate immune system 20.
Quantity of Spike Protein
How much spike is too much? Bansal et al note that Spike protein can be detected circulating on exosomes membranes 4 months post vaccination 58. Virus derived Spike protein has been detected 15 months later in post-acute sequelae patients (PASC or long COVID)38. The vaccine programs have not existed long enough to form a valid comparison in the same tissues. Patterson has recently presented data that both the S1 and S2 segments of spike protein are recoverable from vaccinated persons many months after immunization (personal communication).
mRNA based vaccination has also been shown to provide log scale higher anti-spike antibody production compared to natural infections 59. While some imply this equates to better immunity, it likely comes at the cost of higher spike protein titers and spike protein is believed to be toxic and a potential inhibitor of DNA damage and repair 29.
Estimates of mRNA transfection efficiency have been described by Pardi et al. using a luciferase mRNA 60 transfected into mice. While this does suggest more than 1 protein is synthesized from each mRNA transfected, it is not clear this result can be superimposed onto mRNA derived spike proteins which localize to the nucleus. Quantitative measurement of spike protein expression levels of vaccinated individuals is lacking in the peer reviewed literature.
Toxicity of spike protein
There are multiple modes of spike protein toxicity. Some is attributed to spike protein induced coagulopathy and mitochondrial damage 61. Other toxicity may be a result of Staphylococcus Enterotoxin B (SEB) sequences in the spike protein 62-65.
These SEB motifs share sequence homology to neurotoxic peptides from Cobra’s and are classified as bioweapons 66 67. It should be emphasized that short peptides like the SEB motif may have different properties when cloned into spike proteins and the bioweapon classification has not been applied to the SARs-CoV-2 SEB motif. This motif is unique to SARs-CoV-2 amongst coronaviruses.
Even though the SEB motif is cloned into a different amino acid context, many of the symptoms observed in SEB ingestion are similar to SARs-CoV-2 symptoms as these super antigenic peptides are known for inducing cytokine storms. Cheng et al. suggest SARs-CoV-2 SEB may be responsible for multisystem inflammatory syndrome (MIS-C). Ahanotu et al. describe the symptoms of SEB intoxication as the following
“sudden onset of fever (40-41C), chills, headache, myalgia, non-productive cough. Some patients may develop shortness of breath and chest pain. Fever may last for 2-5 days and cough may continue for up to one month. Patients also present with nausea, vomiting, and diarrhea when the toxin is swallowed.”
The conclusions of Ahanotu et al. are prescient and suggest the most likely method for delivering SEB as a bioweapon would be through the use of an aerosol.
“The use of SEB as a weapon of mass casualty is considered likely for several reasons, mainly high morbidity with ease of production and dispersion, the delayed onset of disease symptoms associated with high morbidity and low mortality and difficulty in diagnosis. Staphylococcal enterotoxin B is a superantigen capable of massive non-specific activation of the immune system. Because of the remarkable toxicity and stability, they would most likely be disseminated as an aerosol”
Finally, the raised GC content and the amplification of G4 quartets in vaccine spike protein mRNAs over the native SARS-CoV-2 spike protein mRNA, can amplify the already established interaction of SARS-CoV-2 RNA with RNA binding proteins11 68. This constitutes a potential cause of interference on epi-transcriptomic regulation of RNA G4 binding proteins. This may play a major role in the potential activation or deactivation of a pathological pathway 69. In this respect, oncogenic RNA binding proteins like the mutant variants of p53 and mdm2 can readily form RNA-Protein binding complexes at polysomes with SARS-CoV-2 vaccine mRNA potential G-quadruplexes 70 71. The vaccine mRNAs prolong their translation due to robust capping resisting natural mRNA decay pathways. This can trigger cancer initiation and progression28.
Conclusions
The argument that the spike proteins synthesized by codon optimized mRNAs are identical to spike protein from the virus should be cautiously examined. There are several arguments that challenge this dogma. First, the biodistribution of non-specific LNP transfection of mRNAs does not discriminate towards ACE2 or CD147 expressing cell lines as seen with the virus. Second, the mRNA that encodes spike protein is known to be different in several regards. The mRNAs are known to have a 2 Proline substitution (K986P and V987P) (Department of Health and Human Services Patent US 10,960,070B2) altering the proteins conformation. The mRNAs are known to be codon optimized thus altering their secondary structure and their quadruplex G density in the spike protein mRNA. The mRNAs are known to have N1-methylpseudourine substitutions that alter translation fidelity and Toll Like Receptor recognition. Additionally, the expression levels and duration of these mRNAs may be longer and of higher copy number in many tissues that never experience natural virus infection. Finally, the pharmacokinetics of injection are different than infection. 60ug-200ug of Spike mRNA equates to 26 Trillion to 80 Trillion mRNA molecules injected in a few seconds. The pharmacokinetics of this bolus injection differs from that of viral replication that occurs over the course of a few days. If each of these mRNAs can produce 10-100 spike proteins and you have 30-40 Trillion cells, there may be a far greater systemic quantity and a much longer duration of spike protein exposure through the vaccination route than natural infection. Boosters given more frequently than a year will lead to total body accumulation of spike protein and further heighten the risk of disease in organs such as the brain, heart, bone marrow, and immune cells and tissues. This false equivalency may lead to an under appreciation of the symptomatology of vaccine based adverse events.
It should be emphasized that these results are an in-silico hypothesis supported by the peer-reviewed literature but further work is required to better characterize the homogeneity of spike protein expression in-vivo. This work has not considered post translational modifications or the impact of the degenerate base pairing from N1-methylpseudouridine.
More than 20 months into this pandemic and we have millions of SARs-CoV-2 genomes sequenced. Lot to lot sequencing of the vaccines is non-existent. To this date, no raw reads for these vaccines exist in NCBI despite over a billion liability-free vaccinations. To fully understand RNA synthesis substitution errors, fragmentation errors or strandedness errors in the mRNA synthesis process, robust lot to lot sequencing should be performed and published. Given these mRNAs are prodrugs which code for a desired protein, where is the evidence that the conversion of this prodrug into a drug is of high fidelity? This seems to have been assumed as opposed to documented. This work suggests this assumption should be questioned. Public and transparent quality control of these often-mandated injections are required. This should include sequence verification and quality control of the various lots and evidence of the proteins these mRNA express in patients.
Acknowledgments. We want to thank Efres Belmonte-Reche for helpful comments.
References
1. Sauna ZE, Kimchi-Sarfaty C. Understanding the contribution of synonymous mutations to human disease. Nat Rev Genet 2011;12(10):683-91. doi: 10.1038/nrg3051 [published Online First: 2011/09/01]
2. Chen R, Davydov EV, Sirota M, et al. Non-synonymous and synonymous coding SNPs show similar likelihood and effect size of human disease association. PloS one 2010;5(10):e13574. doi: 10.1371/journal.pone.0013574 [published Online First: 2010/11/03]
3. Guerra AP, Calvo EP, Wasserman M, et al. Production of recombinant proteins from Plasmodium falciparum in Escherichia coli. Biomedica 2016;36(0):97-108. doi: 10.7705/biomedica.v36i3.3011 [published Online First: 2016/09/14]
4. Denman RB. Using RNAFOLD to predict the activity of small catalytic RNAs. BioTechniques 1993;15(6):1090-5. [published Online First: 1993/12/01]
5. Kikin O, D'Antonio L, Bagga PS. QGRS Mapper: a web-based server for predicting G-quadruplexes in nucleotide sequences. Nucleic acids research 2006;34(Web Server issue):W676-82. doi: 10.1093/nar/gkl253 [published Online First: 2006/07/18]
6. Robinson JT, Thorvaldsdottir H, Wenger AM, et al. Variant Review with the Integrative Genomics Viewer. Cancer research 2017;77(21):e31-e34. doi: 10.1158/0008-5472.CAN-17-0337 [published Online First: 2017/11/03]
7. Thorvaldsdottir H, Robinson JT, Mesirov JP. Integrative Genomics Viewer (IGV): high-performance genomics data visualization and exploration. Briefings in bioinformatics 2013;14(2):178-92. doi: 10.1093/bib/bbs017 [published Online First: 2012/04/21]
8. Robinson JT, Thorvaldsdottir H, Winckler W, et al. Integrative genomics viewer. Nature biotechnology 2011;29(1):24-6. doi: 10.1038/nbt.1754 [published Online First: 2011/01/12]
9. Zhang R, Xiao K, Gu Y, et al. Whole Genome Identification of Potential G-Quadruplexes and Analysis of the G-Quadruplex Binding Domain for SARS-CoV-2. Frontiers in genetics 2020;11:587829. doi: 10.3389/fgene.2020.587829 [published Online First: 2020/12/18]
10. Ji D, Juhas M, Tsang CM, et al. Discovery of G-quadruplex-forming sequences in SARS-CoV-2. Briefings in bioinformatics 2021;22(2):1150-60. doi: 10.1093/bib/bbaa114 [published Online First: 2020/06/03]
11. Cui H, Zhang L. G-Quadruplexes Are Present in Human Coronaviruses Including SARS-CoV-2. Frontiers in microbiology 2020;11:567317. doi: 10.3389/fmicb.2020.567317 [published Online First: 2020/11/17]
12. Belmonte-Reche E, Serrano-Chacon I, Gonzalez C, et al. Potential G-quadruplexes and i-Motifs in the SARS-CoV-2. PloS one 2021;16(6):e0250654. doi: 10.1371/journal.pone.0250654 [published Online First: 2021/06/09]
13. Zhao C, Qin G, Niu J, et al. Targeting RNA G-Quadruplex in SARS-CoV-2: A Promising Therapeutic Target for COVID-19? Angewandte Chemie 2021;60(1):432-38. doi: 10.1002/anie.202011419 [published Online First: 2020/09/18]
14. Hognon C, Miclot T, Garci AIC, et al. Role of RNA Guanine Quadruplexes in Favoring the Dimerization of SARS Unique Domain in Coronaviruses. J Phys Chem Lett 2020;11(14):5661-67. doi: 10.1021/acs.jpclett.0c01097 [published Online First: 2020/06/17]
15. Xia X. Detailed Dissection and Critical Evaluation of the Pfizer/BioNTech and Moderna mRNA Vaccines. Vaccines (Basel) 2021;9(7) doi: 10.3390/vaccines9070734 [published Online First: 2021/08/07]
16. Svidritskiy E, Madireddy R, Korostelev AA. Structural Basis for Translation Termination on a Pseudouridylated Stop Codon. Journal of molecular biology 2016;428(10 Pt B):2228-36. doi: 10.1016/j.jmb.2016.04.018 [published Online First: 2016/04/25]
17. Eyler DE, Franco MK, Batool Z, et al. Pseudouridinylation of mRNA coding sequences alters translation. Proceedings of the National Academy of Sciences of the United States of America 2019;116(46):23068-74. doi: 10.1073/pnas.1821754116 [published Online First: 2019/11/02]
18. Nance KD, Meier JL. Modifications in an Emergency: The Role of N1-Methylpseudouridine in COVID-19 Vaccines. ACS Cent Sci 2021;7(5):748-56. doi: 10.1021/acscentsci.1c00197 [published Online First: 2021/06/03]
19. Kariko K, Ni H, Capodici J, et al. mRNA is an endogenous ligand for Toll-like receptor 3. The Journal of biological chemistry 2004;279(13):12542-50. doi: 10.1074/jbc.M310175200 [published Online First: 2004/01/20]
20. Fohse. The BNT162b2 mRNA vaccine against SARS-CoV-2 reprograms both adaptive and innate immune responses. MedRXiv 2021 doi: https://doi.org/10.1101/2021.05.03.21256520
21. Furer V, Zisman D, Elkayam O. Comment on: Herpes zoster following BNT162b2 mRNA Covid-19 vaccination in patients with autoimmune inflammatory rheumatic diseases: a case series. Reply. Rheumatology (Oxford) 2021 doi: 10.1093/rheumatology/keab702 [published Online First: 2021/09/24]
22. Furer V, Zisman D, Kibari A, et al. Herpes zoster following BNT162b2 mRNA COVID-19 vaccination in patients with autoimmune inflammatory rheumatic diseases: a case series. Rheumatology (Oxford) 2021;60(SI):SI90-SI95. doi: 10.1093/rheumatology/keab345 [published Online First: 2021/04/14]
23. Eid E, Abdullah L, Kurban M, et al. Herpes zoster emergence following mRNA COVID-19 vaccine. J Med Virol2021;93(9):5231-32. doi: 10.1002/jmv.27036 [published Online First: 2021/04/30]
24. Veraldi S, Romagnuolo M, Benzecry V. Pityriasis rosea-like eruption revealing COVID-19. Australas J Dermatol2021;62(2):e333-e34. doi: 10.1111/ajd.13504 [published Online First: 2020/11/21]
25. Veraldi S, Spigariolo CB. Pityriasis rosea and COVID-19. J Med Virol 2021;93(7):4068. doi: 10.1002/jmv.26679 [published Online First: 2020/11/19]
26. Cyrenne BM, Al-Mohammedi F, DeKoven JG, et al. Pityriasis rosea-like eruptions following vaccination with BNT162b2 mRNA COVID-19 Vaccine. J Eur Acad Dermatol Venereol 2021;35(9):e546-e48. doi: 10.1111/jdv.17342 [published Online First: 2021/05/14]
27. Kariko K, Buckstein M, Ni H, et al. Suppression of RNA recognition by Toll-like receptors: the impact of nucleoside modification and the evolutionary origin of RNA. Immunity 2005;23(2):165-75. doi: 10.1016/j.immuni.2005.06.008 [published Online First: 2005/08/23]
28. Kyriakopoulos AM, McCullough PA. Synthetic mRNAs; Their Analogue Caps and Contribution to Disease. Diseases2021;9(3) doi: 10.3390/diseases9030057 [published Online First: 2021/08/28]
29. Jiang H, Mei YF. SARS-CoV-2 Spike Impairs DNA Damage Repair and Inhibits V(D)J Recombination In Vitro. Viruses 2021;13(10) doi: 10.3390/v13102056 [published Online First: 2021/10/27]
30. Walsh EE, Frenck RW, Jr., Falsey AR, et al. Safety and Immunogenicity of Two RNA-Based Covid-19 Vaccine Candidates. The New England journal of medicine 2020;383(25):2439-50. doi: 10.1056/NEJMoa2027906 [published Online First: 2020/10/15]
31. Folegatti PM, Ewer KJ, Aley PK, et al. Safety and immunogenicity of the ChAdOx1 nCoV-19 vaccine against SARS-CoV-2: a preliminary report of a phase 1/2, single-blind, randomised controlled trial. Lancet 2020;396(10249):467-78. doi: 10.1016/S0140-6736(20)31604-4 [published Online First: 2020/07/24]
32. Yousefzadeh M, Henpita C, Vyas R, et al. DNA damage-how and why we age? Elife 2021;10 doi: 10.7554/eLife.62852 [published Online First: 2021/01/30]
33. Hagen T, Laski A, Brummer A, et al. Inosine Substitutions in RNA Activate Latent G-Quadruplexes. J Am Chem Soc2021;143(37):15120-30. doi: 10.1021/jacs.1c05214 [published Online First: 2021/09/15]
34. Jara-Espejo M, Fleming AM, Burrows CJ. Potential G-Quadruplex Forming Sequences and N(6)-Methyladenosine Colocalize at Human Pre-mRNA Intron Splice Sites. ACS Chem Biol 2020;15(6):1292-300. doi: 10.1021/acschembio.0c00260 [published Online First: 2020/05/13]
35. Dae-Eun Jeong MM, Karen Artiles, Orkan Ilbay, Andrew Fire*, Kari Nadeau, Helen Park, Brooke Betts, Scott Boyd, Ramona Hoh, and Massa Shoura*. Assemblies of putative SARS-CoV2-spike-encoding mRNA sequences for vaccines BNT-162b2 and mRNA-1273. GitHub 2021
36. Fernandez IS, Ng CL, Kelley AC, et al. Unusual base pairing during the decoding of a stop codon by the ribosome. Nature 2013;500(7460):107-10. doi: 10.1038/nature12302 [published Online First: 2013/07/03]
37. Drappier M, Jha BK, Stone S, et al. A novel mechanism of RNase L inhibition: Theiler's virus L* protein prevents 2-5A from binding to RNase L. PLoS pathogens 2018;14(4):e1006989. doi: 10.1371/journal.ppat.1006989 [published Online First: 2018/04/14]
38. Bruce K. Patterson EBF, Ram Yogendra, Emily Long, Amruta Pise, Hallison Rodrigues, Eric Hall, Monica Herrara, Purvi Parikh, Jose Guevara-Coto, Timothy J. Triche, Paul Scott, Saboor Hekmati, Dennis Maglinte, Xaiolan Chang, Rodrigo A Mora-Rodríguez, Javier Mora. Persistence of SARS CoV-2 S1 Protein in CD16+ Monocytes in Post-Acute Sequelae of COVID-19 (PASC) Up to 15 Months Post-Infection. bioRxiv 2021 doi: https://doi.org/10.1101/2021.06.25.449905
39. Ram-Mohan. Association Between SARS-CoV-2 RNAemia and Post-Acute Sequelae of COVID-19. MedRXiv 2021 doi: 10.1101/2021.09.03.21262934
40. Katzourakis A, Gifford RJ. Endogenous viral elements in animal genomes. PLoS genetics 2010;6(11):e1001191. doi: 10.1371/journal.pgen.1001191 [published Online First: 2010/12/03]
41. Esnault C, Maestre J, Heidmann T. Human LINE retrotransposons generate processed pseudogenes. Nature genetics2000;24(4):363-7. doi: 10.1038/74184 [published Online First: 2000/03/31]
42. Hu X, Yang L, Mo YY. Role of Pseudogenes in Tumorigenesis. Cancers (Basel) 2018;10(8) doi: 10.3390/cancers10080256 [published Online First: 2018/08/04]
43. Lehrer S, Rheinstein PH. Three Poliovirus Sequences in the Human Genome Associated With Colorectal Cancer. Cancer Genomics Proteomics 2019;16(1):65-70. doi: 10.21873/cgp.20112 [published Online First: 2018/12/28]
44. Hirano M, Noda T. Genomic organization of the mouse Msh4 gene producing bicistronic, chimeric and antisense mRNA. Gene 2004;342(1):165-77. doi: 10.1016/j.gene.2004.08.016 [published Online First: 2004/11/06]
45. Elfman J, Li H. Chimeric RNA in Cancer and Stem Cell Differentiation. Stem Cells Int 2018;2018:3178789. doi: 10.1155/2018/3178789 [published Online First: 2018/12/05]
46. Kury P, Nath A, Creange A, et al. Human Endogenous Retroviruses in Neurological Diseases. Trends Mol Med2018;24(4):379-94. doi: 10.1016/j.molmed.2018.02.007 [published Online First: 2018/03/20]
47. Perron H, Dougier-Reynaud HL, Lomparski C, et al. Human endogenous retrovirus protein activates innate immunity and promotes experimental allergic encephalomyelitis in mice. PloS one 2013;8(12):e80128. doi: 10.1371/journal.pone.0080128 [published Online First: 2013/12/11]
48. Zhang L, Richards A, Barrasa MI, et al. Reverse-transcribed SARS-CoV-2 RNA can integrate into the genome of cultured human cells and can be expressed in patient-derived tissues. Proceedings of the National Academy of Sciences of the United States of America 2021;118(21) doi: 10.1073/pnas.2105968118 [published Online First: 2021/05/08]
49. Kazachenka A, Kassiotis G. SARS-CoV-2-Host Chimeric RNA-Sequencing Reads Do Not Necessarily Arise From Virus Integration Into the Host DNA. Frontiers in microbiology 2021;12:676693. doi: 10.3389/fmicb.2021.676693 [published Online First: 2021/06/22]
50. Liu J, Wang J, Xu J, et al. Comprehensive investigations revealed consistent pathophysiological alterations after vaccination with COVID-19 vaccines. Cell Discov 2021;7(1):99. doi: 10.1038/s41421-021-00329-3 [published Online First: 2021/10/27]
51. Cheng MH, Porritt RA, Rivas MN, et al. A monoclonal antibody against staphylococcal enterotoxin B superantigen inhibits SARS-CoV-2 entry in vitro. bioRxiv 2020 doi: 10.1101/2020.11.24.395079 [published Online First: 2020/12/04]
52. Nielsen HB, Almeida M, Juncker AS, et al. Identification and assembly of genomes and genetic elements in complex metagenomic samples without using reference genomes. Nature biotechnology 2014;32(8):822-8. doi: 10.1038/nbt.2939 [published Online First: 2014/07/07]
53. Penzo M, Guerrieri AN, Zacchini F, et al. RNA Pseudouridylation in Physiology and Medicine: For Better and for Worse. Genes (Basel) 2017;8(11) doi: 10.3390/genes8110301 [published Online First: 2017/11/07]
54. Li X, Zhu P, Ma S, et al. Chemical pulldown reveals dynamic pseudouridylation of the mammalian transcriptome. Nat Chem Biol 2015;11(8):592-7. doi: 10.1038/nchembio.1836 [published Online First: 2015/06/16]
55. Uddin MB, Wang Z, Yang C. Dysregulations of Functional RNA Modifications in Cancer, Cancer Stemness and Cancer Therapeutics. Theranostics 2020;10(7):3164-89. doi: 10.7150/thno.41687 [published Online First: 2020/03/21]
56. Guzzi N, Ciesla M, Ngoc PCT, et al. Pseudouridylation of tRNA-Derived Fragments Steers Translational Control in Stem Cells. Cell 2018;173(5):1204-16 e26. doi: 10.1016/j.cell.2018.03.008 [published Online First: 2018/04/10]
57. Tyson JR, James P, Stoddart D, et al. Improvements to the ARTIC multiplex PCR method for SARS-CoV-2 genome sequencing using nanopore. bioRxiv 2020 doi: 10.1101/2020.09.04.283077 [published Online First: 2020/09/11]
58. Bansal S, Perincheri S, Fleming T, et al. Cutting Edge: Circulating Exosomes with COVID Spike Protein Are Induced by BNT162b2 (Pfizer-BioNTech) Vaccination prior to Development of Antibodies: A Novel Mechanism for Immune Activation by mRNA Vaccines. J Immunol 2021 doi: 10.4049/jimmunol.2100637 [published Online First: 2021/10/17]
59. Gobbi F, Buonfrate D, Moro L, et al. Antibody Response to the BNT162b2 mRNA COVID-19 Vaccine in Subjects with Prior SARS-CoV-2 Infection. Viruses 2021;13(3) doi: 10.3390/v13030422 [published Online First: 2021/04/04]
60. Pardi N, Tuyishime S, Muramatsu H, et al. Expression kinetics of nucleoside-modified mRNA delivered in lipid nanoparticles to mice by various routes. J Control Release 2015;217:345-51. doi: 10.1016/j.jconrel.2015.08.007 [published Online First: 2015/08/13]
61. Lei Y, Zhang J, Schiavon CR, et al. SARS-CoV-2 Spike Protein Impairs Endothelial Function via Downregulation of ACE 2. Circ Res 2021;128(9):1323-26. doi: 10.1161/CIRCRESAHA.121.318902 [published Online First: 2021/04/01]
62. Cheng MH, Zhang S, Porritt RA, et al. Superantigenic character of an insert unique to SARS-CoV-2 spike supported by skewed TCR repertoire in patients with hyperinflammation. Proceedings of the National Academy of Sciences of the United States of America 2020;117(41):25254-62. doi: 10.1073/pnas.2010722117 [published Online First: 2020/09/30]
63. Brown M, Bhardwaj N. Super(antigen) target for SARS-CoV-2. Nat Rev Immunol 2021;21(2):72. doi: 10.1038/s41577-021-00502-5 [published Online First: 2021/01/13]
64. Cheng MH, Porritt RA, Rivas MN, et al. A monoclonal antibody against staphylococcal enterotoxin B superantigen inhibits SARS-CoV-2 entry in vitro. Structure 2021;29(9):951-62 e3. doi: 10.1016/j.str.2021.04.005 [published Online First: 2021/05/01]
65. Bittmann S WA, Luchter E, Moschüring-Alieva, Villalon G. Multisystem inflammatory syndrome in children (MIS-C): The role of
viral superantigens in COVID-19 disease. J Allergy Infect Dis 2020;2020; 1(1):18-20.
Fantastic article!
Two things you might be interested in: (1) the moderna sequence in that paper is flawed because it was sequenced from a degenerate vaccine sample (check the BLAST against WT Wuhan viral AA sequence). The Pfizer sequence is 100% correct (2) The GC content is conspicuously reduced in the furin cleavage site portion of the Pfizer sequence, whereas the original viral sequence is GC-rich in this area. Bizarre eh?
Kevin, I'm trying to understand the good,bad, and ugly side of the G4 formation, from what I'm seeing outside of it as a target with laser like precision for cancers, it's formations from mrna translation or otherwise looks all bad, am I wrong? Also I came across another method for screening for them, don't know if this is an accepted or helpful method. https://academic.oup.com/bioinformatics/article/33/22/3532/4061281